Below is a summary of the abstract you submitted. Presenting author(s) is shown in bold.
If any changes need to be made, you can modify the abstract or change the authors.
You can also download a .docx version of this abstract.
If there are any problems, please email Dan at dar78@pitt.edu and he'll take care of them!
This abstract was last modified on May 1, 2018 at 12:28 p.m..
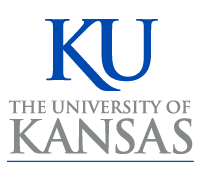
In 2017, the University of Kansas SEA-PHAGE class isolated and characterized Pollux, a temperate bacteriophage that infects Gordonia terrae. Sequence analysis revealed that it is a member of cluster CY1. BLAST analysis of the Pollux genome revealed strong sequence similarity to other CY phages in the left arm of the genome, and blocks of sequence similarity to clusters CV, CZ, DC, DH and DQ in the right arm of the genome. Repressor-based immunity and other prophage defense mechanisms have been extensively studied in mycobacteriophages, whereas similar functions in Gordonia bacteriophages are just being characterized. To investigate immunity of this phage, we generated a Pollux lysogen and infected it with 8 different Gordonia phages. The Pollux lysogen is immune from infection by CY phages and one CZ phage (Ebert), a DH and a DQ phage. Interestingly, one gene that is shared in 5 of the 6 genomes is pham 5013, which encodes a putative immunity repressor, confirming our annotation of that gene and suggesting that all of these immune responses are repressor mediated. Chidebere (a DQ phage) does not share the repressor gene, and has no obvious gene similarities. We would like to test additional phages related to Chidebere in order to identify candidate genes that may mediate this immunity. A second project in the lab sought to identify the integration site of Pollux in the Gordonia genome. BLAST analysis did not reveal a likely AttB site in Gordonia. Therefore, to identify the integration site experimentally, we isolated genomic DNA from the Pollux lysogen, digested it with 10 different restriction enzymes that cut near and on either side of the integrase gene. We diluted the digests, ligated them and performed polymerase chain reaction (PCR) with outward directed primers specific to the integrase gene in order to amplify the boundary between the prophage and bacterial genomes. Thus far we have not succeeded in generating a specific PCR product for sequencing. If we obtain a specific band we will sequence the product in order to identify the AttP and AttB sites in the phage and bacteria. In a third project, we investigated the prevalence of RNA splicing in mycobacteriophages. We queried the actinobacteriophage database for genomes having the same pham in two adjacent genes using SQL. We found over 1600 cases, but these represented only 152 unique instances. Many of these could be eliminated as programmed translational frameshifts. We obtained 8 mycobacteriophages having putative spliced genes, and designed primers spanning the potential intron. We then compared PCR fragment lengths from reactions using either genomic DNA or cDNA that we generated from RNA isolated from 2-hour phage infections of M. smegmatis. Thus far, we have not been able to generate PCR products from the cDNA and so do not have definitive results. However, the low incidence of putative splicing events suggests that splicing is a rare event in mycobacteriophage genes.